The wind
Dr. writes. Nikos Mazarakis
Physicist – Meteorologist
Wind is perhaps the most important meteorological phenomenon that affects our activities at sea, as it is the wind that shapes the height of the waves and the general state of the sea. Atmospheric air can move in two ways either horizontally or vertically. By definition wind is the horizontal movement of atmospheric air. The vertical movements of atmospheric air are what shape the weather conditions in a region, so to speak. So the upward motion is what contributes to the creation of clouds and the opposite is the downward motion. In this article we will see in more detail the horizontal movement of atmospheric air.
Cause of wind generation
The main cause of creation of the wind is its force weight scale, which is due to the fact that the barometric pressure is not the same in every area of the earth's surface. The force of the barogradient has a direction perpendicular to the isobars and always goes towards the low pressures, as can be seen in figure 1. So if only this force was exerted on the atmospheric air, the wind would always have a direction from the barometric highs (anticyclones) to the barometric lows (cyclones). However, this does not actually happen, as an additional force is exerted on the air mass, which is due to the rotation of the earth and is called the Coriolis force, in honor of the French mathematician and engineer Gaspard-Gustave Coriolis who was born in Paris in 1972 and contributed significantly to study of motion in rotating reference frames.
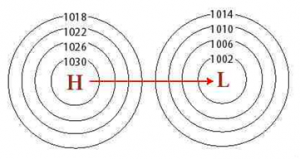
THE Coriolis force it results in bodies moving in the atmosphere being deflected to the right in the Earth's northern hemisphere (N.H.) and to the left in the southern hemisphere (see figure 2). The Coriolis force depends on the latitude of a place and is zero on the equator. It is the main force causing the rotational motion in the lows, which is counter-clockwise in B.H. and according to the hands of the clock in N.H. The Coriolis force is also proportional to the speed of the moving body. So when a body is stationary, this force is not exerted on it.

As shown in figure 3, if we leave an air mass at point A, then it will spontaneously start moving towards low pressures, due to the force of the barogradient. But as the speed of the air mass increases, so will the Coriolis force which is always perpendicular to the speed of the moving body. This has the result that after a certain distance the Coriolis force becomes equal but opposite in direction to the barogradient force. Thus the total force exerted on the air mass (consistency) will be zero. But in our school years we learned 2The Newton's law, which says that when no forces are exerted on a body or they are exerted but their component is zero, the body will remain stationary or move in a straight line at a constant speed.

In the atmosphere the second happens, i.e. the atmospheric air eventually moves parallel to the isobars having to its left the low pressures in B.H. and on his right in N.H. This wind is called geostrophic wind and it is, as we have seen, the result of only two forces, the barogradient and the Coriolis. The geostrophic wind always blows above 500 meters altitude, as on the surface and up to the height of 500 meters, a third force appears, which is none other than our familiar one rubbing.
Friction is a force due to the relative movement of atmospheric air over the earth's surface. Friction always has the same direction as the velocity of the body and direction opposite to the velocity. Friction depends on the topography of the ground. The rougher the ground, the greater the force of friction. Friction has its lowest value above the flat sea, while above the ground it is difficult to calculate it due to the diversity of the relief (presence of vegetation, constructions, etc.). As can be seen in figure 4, the final balance of these three forces results in the wind on the surface not being parallel to the isobars but always having a slope towards low pressures. This inclination varies between 10 and 30 degrees over the sea, where we have the least friction, while over land it can even reach 45 degrees. This explains the fact that when we are on an island on the coast the wind intensity is much stronger on the coast than in the interior of the island.

Friction is greater in the surface layers, while as we go up the force continuously decreases and becomes zero at about 500 meters altitude. Thus, as can be seen in Figure 5, the wind, while on the surface it intersects the isobars always towards the low pressures with an angle that, as we said, ranges from 10 to 45 degrees, going up this angle continuously decreases and at 500 meters it becomes zero with result in the wind moving parallel to the isobars and increasing in intensity.

In simpler terms, the direction and intensity of the wind is different at the surface than it is high in the atmosphere. We have all noticed that clouds move at a higher speed and in a different direction than the wind at the surface. For example, if we have northerly winds on the surface and the clouds are at a height of 500 – 1000 meters, then we will see them moving to the southwest and not to the south. The variation and intensity of the wind is even more noticeable as we climb higher in the atmosphere. Thus approximately in the middle of the troposphere, the layer of the earth's atmosphere where all meteorological phenomena develop with an average thickness of 12 km, the wind almost always has a westerly direction in both hemispheres of the earth and typical speeds that can even reach 150 knots . The westerly direction of the wind is basically due to the rotation of the earth and is the reason that almost all weather systems have a movement from west to east. However, the prevailing westerly direction of the wind in the upper atmospheric layers is also the reason why the duration of air travel is shorter when the aircraft moves from west to east than the opposite, since in the second case the wind is against it.
Wind intensity
Surface wind intensity can be determined relatively accurately by numerical weather prediction models, but only over the sea, where the frictional force can be accurately estimated. Above land, however, the interaction of the atmospheric air with the relief acquires chaotic dimensions, with the result that the weather forecast models are not able to accurately simulate the wind field and thus the forecast is unrealistic. In simpler words when looking at the wind forecast on the internet, we should not give any weight to the wind forecast over land, only over sea. Over land the wind can be accurately predicted, but the resolution of the weather model must be very high, which in operational forecasting is unfeasible.
On surface maps the wind intensity is determined solely by the distance of the isobars, which in most cases are plotted every 4 mb. The shorter the distance between the isobars, the stronger the wind between the isobars. A practical rule for the Aegean sea is the following: If you see on a map that there are more than 4 isobaric curves in the Aegean sea area in the Thessaloniki-Rhodes straight line, then the wind intensity is more than 7 mph. (see figure 6). Another also practical rule that I like to teach my students is this: imagine that the isobars are like the banks of a river. We know that in a river, the closer the banks get, the faster the water becomes. Something similar happens with the wind, if we put the isobars in the place of the river banks and the wind in the place of the water. The only difference is that while the water flows parallel to the banks, the wind forms as we said before, a small angle with the isobars, always looking towards the low pressures.

Time for practice
Taking into account all of the above, looking at a surface map and looking at the isobars, we can make a rough estimate of the direction and the intensity of the wind. In figure 7 we see such an example. As we said earlier in B.H. the wind always blows to the left of the low pressures and forms an angle of 10 to 30 degrees to the low pressures over the sea, with the angle increasing as the wind intensity increases. So in relation to the position of the low in the South Ionian, shown on the UK Met Office's 24 hour forecast map (source: http://www.cincfleetwoc.com/HF-Fax/North_Atlantic/matrix.html#matrixLatestZData), with an effective time and date of 1800 UTC, 3 June 2014 (+3 hours local time), the wind in Crete will be southeasterly, gradually turning to east-northeasterly as we head towards the Central Aegean. In the Ionian it will be north-easterly. Regarding intensity, we see that in the lower right part of the map there is a scale, called geostrophic wind scale. This scale helps us to make a rough estimate of the wind intensity as follows: With a compass or a simple ruler we measure the distance (opening) of the isobars to the point we want (red line). We then transfer this distance to the lower right diagram, placing the left part of the red line at the latitude of our area. The right edge of the red line shows us the intensity of the geostrophic wind, which in our example is about 38 knots. However, the intensity of the actual wind on the surface is equal to 2/3 of the geostrophic and thus the intensity on the surface will be approximately equal to 25 knots. In reality, however, this intensity can be significantly lower than the real one, as due to the special geomorphological characteristics of our country, the wind can be significantly strengthened, depending on the direction and in combination with the topography of an area.
